PRB 01-4E
OZONE: THE
EARTH'S SUNSCREEN
Prepared by:
Joanne Agterberg, Daniel Brassard
Science and Technology Division
2 April 2001
TABLE OF CONTENTS
INTRODUCTION
THE
ALTERED NATURAL SYSTEM
A. Thermal Radiative
Balance
B. Ultraviolet Rays
1. Impact on Human Health
2. Impact on Terrestrial
Flora
3. Impact on Aquatic Life
4.
Impact on Air Pollution
EXTENT OF THE DAMAGE
OZONE-DEPLETING
SUBSTANCES
A. Agents
B. Innovation
ACTION TO REDUCE THE DESTRUCTIVE AGENTS
A. International Action
B. Federal Initiatives
C. Federal-Provincial
Action
D. Action Still to be Taken
SUMMARY AND CONCLUSION
APPENDIX:
OZONE-DEPLETING SUBSTANCES
OZONE: THE EARTH'S
SUNSCREEN
INTRODUCTION
Life on Earth is a delicate balance of
many different elements. The conditions necessary for the diversity and abundance of
life are fairly restricted and any major change can have unpredictable results. The
energy of the sun maintains all forms of life on the planet.
Ultraviolet radiation (UV) is one of the
main components of the sun’s energy transmitted to the Earth. This radiation is
necessary in moderation; in excess, it can cause serious problems for humans and the
biosphere in general. Ozone in the stratosphere moderates the amount of radiation
reaching the Earth. The stratosphere is a layer of the atmosphere above the lower
layer and denoted by a temperature inversion where ozone acts as the Earth’s
sunscreen, filtering out the most harmful wavelengths of UV.
Since the 1970s, we have known that
various manufactured products are destroying this life-preserving filter. Findings
by the U.S. National Aeronautics & Space Administration (NASA) suggest that upper
atmospheric ozone loss in the North will likely continue to increase in the coming years.(1) According to several scientific
models and a report issued by Environment Canada, serious depletion episodes could become
more frequent over the next 20 years, with the most frequent ozone destruction in the
Arctic estimated to occur between 2010 and 2020.(2) This paper will discuss the various aspects of the
ozone situation, from technical explanations to the latest efforts to remedy the problem.
THE ALTERED
NATURAL SYSTEM
Over geological time periods, the
atmosphere has changed, becoming the Earth’s protective blanket, enabling life to
exist. Life on Earth evolved slowly so that each portion of the ecosystem is adapted
to make best use of the available conditions. Over the past century, human activity
has altered the atmosphere at an unnaturally rapid rate, thereby reducing the ability of
some organisms to adapt.
Although certain modifications to our
surroundings are apparent, others are more difficult to observe. Smog, for instance,
is very noticeable; however, the altered absorptive and reflective qualities of the
atmosphere are more difficult to observe directly.
A basic understanding of the processes
surrounding this phenomenon is needed to fully appreciate the importance of a reduced
quantity of ozone in the upper atmosphere. Ozone (O3) is a
pungent-smelling, slightly bluish gas, with one more oxygen atom than molecular oxygen (O2).
It can be found at ground level as pollution, where it is harmful to life, and naturally
up to an altitude of about 60 km (see Figure 1). Generally, 85% of all ozone is
contained in a region of the stratosphere, of varying altitude depending on latitudinal
position, known as the ozone layer.(3)
On average, the amount of global ozone in the stratosphere is 300 Dobson Units (DU),
equivalent to a thickness of pure ozone of only 3 mm, at ground level pressure and
0°C. When stratospheric ozone falls below 200 DU, this is considered the beginnings
of an “ozone hole.”
Figure 1
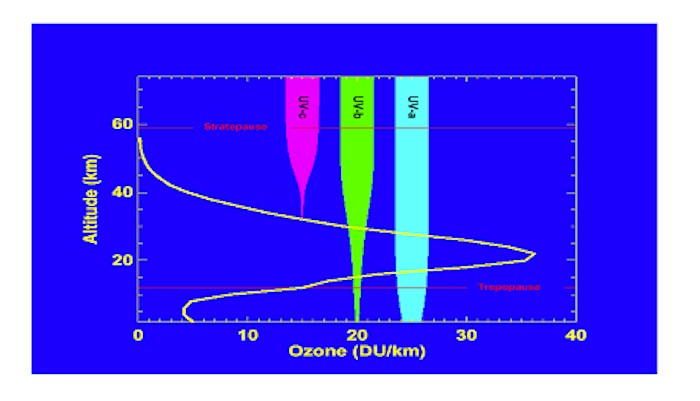
A typical vertical profile of ozone
density in the midlatitudes of the Northern Hemisphere (units=Dobson
Units/kilometre). The stratosphere lies between the tropopause and
stratopause. Superimposed on the figure are plots of UV radiation as a function of
altitude for UV-a (320-400 nm), UV-b (280-320 nm), and UV-c (200-280 nm). The width
of the bar indicates the amount of energy as a function of altitude. The UV-c energy
decreases dramatically as ozone increases because of the strong absorption in the
200-280 nm wavelength band. The UV-b is also strongly absorbed, with a small
fraction reaching the surface. The UV-a is only weakly absorbed by ozone, with some
scattering of radiation near the surface.(4)
Ozone is produced when high-energy
radiation, such as ultraviolet radiation, causes oxygen molecules to split into two oxygen
atoms which recombine with other oxygen molecules. The stratospheric concentration
of ozone reaches new equilibrium points based on the relative rates of its formation and
destruction, which are dependent on various conditions. The greatest ozone losses
occur at the altitudes where ozone is most abundant, as can be seen in Figure 2.(5)
Figure 2 (6)

The destruction of stratospheric ozone is
complex and involves numerous factors. The process is accelerated by the presence of
significant levels of catalysts,(7)
specifically chlorine and bromine, members of the extremely reactive “halogen
family” of elements. The chlorine and bromine in the stratosphere originate
from numerous sources, both man-made and natural. The major source of halogens in
the atmosphere is man-made products known as halocarbons,(8) which constituted 82% of chlorine entering the
stratosphere in the early 1990s.(9)
Natural sources of halogens have a shorter lifespan than halocarbons. Unless
injected directly into the stratosphere by major volcanic activity, they are dissolved and
washed out by precipitation found in lower regions of the atmosphere where weather is
prevalent. Halocarbons do not dissolve in water and therefore all emissions
eventually reach the stratosphere via atmospheric mixing, typically over a period of five
years.
When halocarbons reach the stratosphere,
high-energy ultraviolet radiation and contact with other chemicals break the bonds that
hold the atoms together, liberating the halogens to chemically bond in several other ways,
as hydrogen chloride or chlorine nitrate, for example.
The stratosphere is known for its absence
of clouds, yet ozone depletion occurs most often on polar stratospheric clouds (PSCs),
luminescent clouds consisting of super-cooled water, nitric and sulphuric acid. PSCs
form at low temperatures and act as active sites(10) on which a very slow reaction in the gas phase can go
very quickly heterogeneously, i.e., on solid surfaces. When PSCs occur, the chlorine
which is sequestered, by hydrogen ions(11)
or nitrogen oxides,(12) is liberated
as chlorine gas (Cl2), producing nitric acid.(13) Several other heterogeneous reactions also take place on
PSCs, contributing to ozone depletion.
The molecular chlorine is dissociated by
solar radiation when polar night has ended. Chlorine atoms react with ozone (O3)
to form chlorine monoxide (C1O) and molecular oxygen (O2). Chlorine
monoxide then combines with atomic oxygen (O), produced by the process of natural ozone
creation, to produce molecular oxygen (O2) and regenerate atomic chlorine
(Cl). Typically, one chlorine atom, acting as a catalyst, can destroy up to 100,000
ozone molecules, repeatedly destroying ozone molecules, when conditions are right, for
about 100 years.
Polar regions experience the greatest
number of PSCs due to a phenomenon known as the polar vortex. This rotating parcel
of extremely cold stratospheric air occurs during the long polar night. The vortex
prevents atmospheric mixing of additional stratospheric ozone or heat. Rapid ozone
loss occurs on these platforms when sunlight returns to photolyse(14) the chlorine molecules. Often PSC particles
containing nitrogen will grow in size(15)
and settle to a lower level, known as denitrification. Therefore, the chlorine does
not become sequestered by nitrogen oxides again but rather a continuation of catalytic
ozone destruction occurs.(16)
Because the topography of the Antarctic is
generally uniform, the southern polar vortex is stronger than at the Arctic. In
localized areas over Antarctica, ozone can drop dramatically by up to 70% in the course of
a few weeks.(17)
Environmental consequences of ozone
depletion are two-fold. The earth’s radiative energy balance is altered and the
amount of harmful ultraviolet solar rays reaching the Earth’s surface
increases.
A. Thermal Radiative
Balance
Ozone plays a role in regulating the flow
of heat through the atmosphere by absorbing the sun’s radiation. The exact
distribution of ozone in the stratosphere thus affects the temperature of the atmosphere
and weather patterns. Even small changes in the energy input to the atmosphere from
the sun or the energy exchange by radiation between the ground and the atmosphere can
influence climate.(18) The
temperature distribution in the stratosphere is directly dependent on the distribution of
ozone.(19) This is of great
consequence because ozone depletion is highly sensitive to temperature.
B. Ultraviolet
Rays
The sun emits a wide spectrum of
energy. Some is in the form of ultraviolet energy, which has a wavelength shorter
than violet light. The portion of the ultraviolet light with wavelengths from 280 to
320 nanometres (or 10-9m), known as ultraviolet B (UV-B), is dangerous in
excess. UV-C rays are even more energetic, but they are almost fully filtered out by
ozone. A decrease in the ozone layer increases UV-B intensity at ground level by
approximately twice the percentage decrease, other factors excluded. For example,
with an ozone decrease of 30%, UV-B reaching Earth’s surface increases by 60%.(20)
High levels of UV-B cause synthetic
materials such as plastic, as well as natural materials including wood, to undergo
increased rates of discolouration and weakening. Many classes of materials may be
susceptible; research is ongoing.
1. Impact on Human Health
Ultraviolet light can break the chemical
bonds in various substances including DNA, the molecules that encode genetic
information. The effects of UV radiation are complex and difficult to interpret, but
several consequences of increased levels of UV-B on humans have been confirmed.
Excess exposure to UV-B radiation,
especially in childhood, causes higher rates of skin cancer. One reason for this is
that UV-B suppresses the skin’s immune responses, so that the body is less likely to
reject a growing tumour.(21) A
10% decrease in total stratospheric ozone is expected to cause an additional 300,000 cases
of non-melanoma and 4,500 melanoma skin cancers globally each year.(22) Without the Montreal Protocol (discussed below in
section entitled “A. International Action”), ozone depletion would have
accounted for an additional 20 million cases of skin cancer.(23)
Because ultraviolet light suppresses the
immune system, particularly in skin cells, infectious diseases having a stage involving
the skin are likely to increase. Also, the effectiveness of vaccines against
infectious disease may be limited.(24)
Repeated exposure to excessive UV
radiation also sensitizes the eyes to developing cataracts, the main cause of blindness in
the world. An estimated 20% of cataracts is due to UV exposure.(25) Other confirmed effects include age-related
nearsightedness and deformation of the lens capsule.
To reduce health risks, individuals can do
a number of things such as applying a sunscreen with a sun protection factor of at least
15, wearing hats and protective clothing, staying in the shade as much as possible, and
wearing high-quality sunglasses treated to absorb UV-B radiation. With proper
precautions, the negative health effects of ozone reduction can be minimized.
2. Impact on
Terrestrial Flora
The increased exposure to ultraviolet
radiation may have a dramatic effect on plant life by affecting hormones and growth.
Observed specific effects include:
a reduction in total dry weight,
nutrient content, and area available for photosynthesis;
deterioration in the quality of certain
types of tomato, legume, cabbage, potato and sugar beet;
diminished flowering/germination rates;
and
distribution of nutrients within plants.
While some crops, such as soybean, show
decreased production as a result of elevated amounts of UV-B, many other crops are
unaffected, or show a high degree of tolerance. Research indicates that the growth
and photosynthesis of certain plants (e.g., seedlings of rye, maize and sunflower) can be
inhibited even under ambient levels of UV-B.(26)
For many organisms, protective mechanisms
may not be sufficient to protect against increased levels of UV radiation. (There
are indications that some weeds are more UV-resistant than crops.) Exposure tests
have shown that more than 100 species of land plants could be sensitive to increases in UV
radiation as a result of stratospheric ozone depletion. International research has
revealed that some species of rice suffer from even minor increases in UV-B.
Research into the efficient breeding and cultivation of strong species may help to offset
some of the damaging effects.
Because the sensitivity of trees and other
plant species varies, the diversity and distribution of such species could change, and it
is even possible that some species could disappear.(27) Studies show that many species of conifer are
negatively affected by increased UV-B radiation.
An increase in the natural level of UV-B
is known to cause mutations in microorganisms. However, with the possibility of more
rapid mutations, rapid adaptation to UV-B stress may result. Although some research
is showing that limited adaptation is possible, most of the effects remain very
negative. A decreased rate of the fixing of atmospheric nitrogen by fungi would
require significant global substitution by artificial fertilizer.(28) Nitrogen compounds, largely produced by
microorganisms in the soil, seem to play a major role in the natural control of ozone
levels.(29)
3. Impact on Aquatic Life
All areas of the biosphere appear to be
affected by increased UV-B to some extent, some more so than others. Phytoplankton
(phyto = plant) is highly sensitive to UV radiation; it lacks the protective layers of
higher forms of life and drifts along at the water’s surface where exposure to UV-B
can occur. As well as being the basis of the aquatic food web, phytoplankton is a
major oxygen producer and a carbon sink, known to assimilate large amounts of atmospheric
carbon dioxide. Marine phytoplankton produces at least as much biomass as all
terrestrial ecosystems combined. Changes to phytoplankton production could
exacerbate the greenhouse effect.
Scientists at the University of Plymouth
have found that the reproductive cells of algae are several times more sensitive to
ultraviolet radiation than are mature cells. Laboratory research revealed that
asexual spores of the selected species were six times more sensitive to UV-B than were
mature plankton. Free-swimming gametes, the sexual means of reproduction, were even
more susceptible. Ecological impact could be greatest at particular times in the
spring, during so-called “blooms” when plankton reproduction is most intense.(30) Assessment of these effects
on phytoplankton is difficult because of the lack of data describing UV-B and biological
levels during the period before the depletion of the ozone layer.
Zooplankton (zoo = animal) – small
organisms, such as fish and crustacean larvae – are at risk as well as anchovies
because they spend critical periods near the surface of the water where UV-B can
penetrate. A reduction of these organisms found near the base of the food chain
could result in a reduction of the fish population. This may have an adverse impact
on the global food supply. Fish account for 18% of the animal protein in the world
and 40% of the protein consumed in Asia.(31)
Freshwater marine ecosystems are also at risk from the intensification of UV-B exposure.(32)
There is widespread agreement that
ozone-related increases in UV-B have the potential to cause wide-ranging direct and
indirect effects on aquatic ecosystems, especially at the poles where life forms are less
acclimatized to intense sunlight. Studies generally show that the increased UV
radiation under the Antarctic ozone hole reduces plankton production by between 6% and 12%
during exposure. Damage at the molecular, cellular, population and community levels
has been demonstrated.
4. Impact
on Air Pollution
Atmospheric changes resulting from the
increased UV-B radiation are of special concern. Diminished air quality in the
troposphere, the lower level of the atmosphere, has been noted. Chemical reactivity
in the troposphere increases in response to increased UV-B, resulting in higher levels of
air pollution. UV-B stimulates the formation of molecules that react rapidly with
other chemicals to form new substances. For example, hydroxyl radicals are
generated, which contribute to the creation of ground level ozone, aerosols and other
harmful pollutants. In urban areas, a 10% reduction of the ozone layer is likely to
result in a 10-25% increase in tropospheric ozone. Irradiated smog contributes to
oxidized organic(33) chemicals, such
as formaldehydes. These molecules tend to form reactive hydrogen radicals, which
contribute to the formation of hydrogen peroxide. Hydrogen peroxide is the principal
chemical that oxidizes sulfur dioxide to form sulfuric acid in cloud water, making it an
important part of acid rain formation.(34)
EXTENT OF THE DAMAGE
Increasingly severe ozone depletion
now occurs each spring over Antarctica (see Figure 3), and the year-round levels are
lower than normal. The year 2000 ozone hole was the largest ever over Antarctica,
forming two weeks earlier than before and extending past the polar vortex.(35)
Figure 3 (36)
Arctic ozone depletion has
not occurred as dramatically as over the Antarctic. The Arctic region’s polar
vortex usually dissipates in late winter before sunlight returns. However,
occurrences of significant ozone depletion are becoming more frequent in the Arctic.
Many international projects involving satellites, high altitude aircraft, balloons and
ground stations are helping to improve understanding of processes that govern the
generation, depletion and distribution of ozone. A variety of techniques are used to
detect and measure the various factors involved. NASA’s Upper Atmosphere
Research Satellite (UARS) was launched in September 1991. This satellite was
configured to measure levels of ozone-depleting chemicals as well as ozone levels at
various altitudes. The U.S. TOMS (Total Ozone Mapping Spectrometer) satellite is
used to deduce the ozone levels from the back-scattered sunlight in the UV range.
The Global Ozone Monitoring Experiment
(GOME), launched in April 1995 on the European ERS-2 satellite, marked the beginning of a
long-term ozone monitoring effort. Scientists receive high-quality data on the
global distribution of ozone and several other climate-influencing trace gases in the
Earth’s atmosphere. GODIVA is the Global Ozone Data Interpretation, Validation
and Application component of GOME, which also contributes to other experiments such as the
Third European Stratospheric Experiment on Ozone 2000 (THESEO 2000) and the SAGE III Ozone
Loss and Validation Experiment (SOLVE) which have been conducted jointly. More than
350 researchers have participated from the U.S., Europe, Russia, Japan and Canada.
Project results support the theory that recovery of the ozone layer may be delayed due to
a strengthening of the polar vortex and a cooling of the stratosphere.
Environment Canada is involved in a
variety of experiments dealing with ozone chemistry, such as the Canadian Middle
Atmosphere Initiative, with the purpose of modeling, assimilating data, and monitoring
this region of the atmosphere.(37) A
Canadian satellite (SCISAT-1) of the Atmospheric Chemistry Experiment (ACE) is expected to
be launched in 2002 to study global ozone depletion.(38)
Analysis of satellite data reveals that
the loss of ozone in the Northern Hemisphere is now proceeding faster than previously
thought. Although no Arctic ozone losses comparable with those in the Antarctic have
occurred, ozone losses have increased greatly during the 1990s in the Arctic.
Isolated ozone losses approaching 45% give cause for concern due to the higher number of
human populations than at the South Pole.(39)
At both poles, ozone loss is expected to
grow larger during the coming decades before recovering. It has been recently
discovered that greenhouse gases are causing more dramatic upper-atmospheric cooling.(40) Computer models predict that
temperature and wind changes induced by greenhouse gas emissions may allow a stronger and
longer-lasting atmospheric vortex to form above the Arctic.
OZONE-DEPLETING
SUBSTANCES
A. Agents
When concern about the ozone layer first
surfaced in the 1970s, attention initially focused on advanced aircraft as the root of the
problem, then shifted to the ordinary aerosol spray can. The group of industrial
chemicals known as CFCs (chlorofluorocarbons) were used as propellants at that time.
Thousands of tonnes were being released directly into the lower atmosphere where they
began their gradual drift upward to destroy the stratospheric ozone. Since then, the
list of materials known to destroy the stratospheric ozone has greatly expanded.
The impact of aircraft on stratospheric
ozone depletion is still being researched.(41)
Studies have shown that improved efficiency and management strategies are required to
reduce aviation’s impact on ozone depletion.(42) In-flight measurements of emissions
were taken of supersonic exhaust, greatly contributing to the reliability of studies
regarding the effect of this form of transportation on stratospheric ozone.(43) Emissions of nitrogen
compounds (NO and NO2) from supersonic aircraft is of concern, due to the inevitability of
increasing fleet size and the high altitudes of flight.
CFCs, and the other ozone-destroying
agents, are ubiquitous in almost every society. They are used in a wide range of
products that are frequently not recognized as containing ozone-destroying
materials. Because of the range of materials involved, the term
“ozone-depleting potential” (ODP) was adopted to indicate the potential of a
substance to destroy ozone, on a mass-per-kilogram basis, compared to an ODP value of one
given to Trichlorofluoromethane, known as CFC-11 in the conventional system of
classification. The ODP is based upon various factors such as the substance’s
atmospheric lifetime, molecular weight, its ability to be photolytically disassociated,
and other factors determined to provide an accurate estimate of the relative
ozone-depletion potential.
The table in the Appendix lists many
ozone-destroying agents with associated ODP, as well as some replacements for CFCs.
The hydrochlorofluorocarbons (HCFCs), the current replacement for CFCs, have a low ODP,
while the hydrofluorocarbons (HFCs) have a zero ODP and so are not included. Ongoing
research causes some ODP values to be updated over time. Lifespans are still being
measured. UNEP rates a country’s progress in meeting goals by measuring
production of groups of ozone-depleting substances in ODP tonnes (metric tonnes times
ODP).
Quantitatively, CFCs have been the main
ozone-destroying agents. Because of their stable, non-toxic, non-flammable and
non-corrosive characteristics, they have been used in refrigeration, aerosols, solvents,
and the production of foams. Alternative technologies have been developed for many
of these applications, and the current challenge is to ensure that the vast existing
amounts of CFCs used in refrigeration and air conditioning are reclaimed rather than
permitted to escape into the atmosphere.
The other major group of ozone-destroying
agents are the halons, which are of similar composition to CFCs but contain bromine.
Halons are used as fire-fighting chemicals for numerous reasons, including their low
toxicity, unobstructed visibility during discharge, low corrosive or abrasive residue, and
high effectiveness per pound. Although the production of halons in developed
countries has been largely phased out since 1994, the stratospheric concentration of these
potent ozone destroyers is still rising, due to their long atmospheric lifetimes and the
time required for atmospheric mixing. In developing countries, there are no
restrictions on halons until January 2002. Halons have an extremely high
ozone-depleting potential (three to ten times higher than CFCs), and their intended use
results in release directly to the atmosphere. The remaining agents – such as
carbon tetrachloride, methyl chloroform, and methyl bromide – have been used in
several ways, for example, as feedstocks to produce CFCs, in pesticides and in dry
cleaning agents.
In 1997, the overall halocarbon burden in
the stratosphere, or effective equivalent chlorine (EECL), began to decrease, largely due
to the phasing out of trichloroethane, a cleaning solvent. Due to its relatively
short lifespan of 4.8 years, it is now largely absent from the stratosphere. Without
this influence, the EECL would still be rising; however, the EECL may not decrease
significantly more unless emissions of potent, longer-lasting ozone-depleting substances
(ODS) decline further by 2010. The chemical currently contributing the most to EECL
in the atmosphere is halon-1211. Amounts have remained relatively constant in the
stratosphere despite limitations on its production in developed countries.(44)
B. Innovation
The need to reduce the use of
ozone-destroying agents has stimulated many technological innovations and the use of
alternative materials for each application. Noteworthy innovations include new
propellants, solvents, foaming agents, fire suppressants, and pest management techniques.(45) The urgent need for change
has also made progress in recovery and recycling technology indispensable. The
largest and most difficult changes have taken place in refrigeration and air
conditioning. Most of the research has been aimed at finding alternatives to CFCs as
a refrigerant.
CFCs have been largely phased out in
developed countries. Many replacements have been based on HCFCs and to some extent
on HFCs. The first use of HFC-134 in automobile air conditioners was in 1991, but
its use is quickly expanding. One of the problems associated with the use of HCFCs
and HFCs is that they are less energy efficient than CFCs. DuPont, the world’s
largest producer of CFCs, halted CFC production in 1993, five years earlier than
originally planned, and now produces HCFCs and HFCs. HCFCs are very strong
greenhouse gases, designated by amendments to the Montreal Protocol to be phased out by
2030 in developed countries. Some HFCs also have a large global warming potential
and long lifetimes.
An exciting advance towards resolving the
refrigerant problem is the development of the thermoacoustic refrigerator, which has no
moving parts and uses environmentally benign gases. Although the principle on which
it works is simple, the actual design is complex. A loudspeaker at the end of a tube
produces a loud note that resonates inside a gas-filled tube. This generates a
standing wave,(46) which effectively
transmits the heat towards the antinode.(47),(48) Because this vibration is
accurately controlled, thermoacoustic refrigerators will be quieter than conventional
refrigerators. A recent demonstration at the Los Alamos National Laboratory showed
how the design could be simplified by eliminating the cold heat exchanger and cooling the
thermoacoustic working gas instead.(49)
Another exciting innovation is the
magnetic refrigerator, developed by Astronautics Corporation of America under a contract
with the U.S. Department of Energy. It has comparable energy efficiency to
conventional household units. The cost of the gadolinium magnet is the major
obstacle to its widespread use, although in the near future it will likely be marketed to
large-scale commercial enterprises.(50)
Other solutions include designs with less
coolant but larger surface area coverage of the coolant. An inexpensive yet useful
alternative for desert living recently earned Mohammed Bah Abba of Nigeria a Rolex Prize
for enterprise. The system, based on the cooling properties of evaporation, uses
damp sand between clay pots.(51)
Ionizing radiation is also an alternative to refrigeration for preserving food supplies.(52)
Ammonia is widely used as a refrigerant in
industry, for cold storage warehouses and petrochemical facilities, as examples. The
shortcomings of this refrigerant are its corrosive and flammable nature at certain
concentrations in air. Accidental releases, which are exacerbated by the pressurized
nature of this refrigeration system, have caused injury and death from inhalation of the
fumes and have contaminated water.(53)
In terms of the ozone layer, ammonia would form other stable compounds before reaching the
stratosphere.
Solutions to CFC usage in the production
of foams, for uses ranging from insulation to foam cups, have included lessening the
amount of propellant required and replacing the CFCs with less-damaging HCFCs. The
Lily Cup Inc., of Toronto, has developed an innovative technique after many years of
research, which uses oxygen and recycled carbon dioxide as a foaming agent.(54)
Changes have been made even in the health
care sector. For example, hospitals have reduced the use of CFCs as
sterilants. Canada is phasing out CFC propellants in metered-dose inhalers (MDIs) by
2005. The first CFC-free MDI was approved for use in August 1997. In this
product, the CFC propellant has been replaced by hydrofluoroalkane (HFA), which does not
cause ozone depletion. The safety of HFA for medicinal use has been demonstrated
through extensive testing. The CFC-free MDI has now been approved for use in more
than 35 countries.(55)
The electronics industry was one of the
principal users of CFC-based solvents. For most applications, new solvents that do
not use CFCs have been developed. In 1991, Northern Telecom developed and
implemented an alternative technology for soldering electronic circuit boards which did
not involve needing to use solvents first.(56)
The company co-founded the Industry Co-operative for Ozone Layer (now the International
Co-operative for Environmental Leadership), which shares new technology. This earned
Northern Telecom the Financial Post 1993 Appropriate Technology Award.(57) Many large electronic
manufacturers have now eliminated the use of CFCs.
Heat treatment, the use of diatomaceous
earth, or live biological control are alternatives to the use of methyl bromide as a
produce and crop fumigant. Knowzone Solutions Inc., of Canada, recently acquired the
rights to the Bromosorb® Technology for the capture of methyl bromide after fumigation.
Securiplex Inc. of Canada has developed
several alternatives to halons in fire protection, which rapidly extinguish fires without
equipment damage.
ACTION
TO REDUCE THE DESTRUCTIVE AGENTS
A. International
Action
The international community has taken up
the challenge of halting the destruction of the stratospheric ozone layer. This is
one of the main reasons for the rapid technological development of alternatives to
CFCs. Canada, a very active participant in international discussions, took early
action. In March 1980, this country prohibited the use of CFCs in most common
consumer aerosols, such as hairsprays, deodorants and antiperspirants.
In 1985, the Vienna Convention for the
Protection of the Ozone Layer called for voluntary measures to reduce emissions of
ozone-depleting substances.(58)
Canada was the first nation to ratify the Vienna Convention in June 1986. More
comprehensive initiatives have been agreed to since this first major international
agreement.
In an unprecedented demonstration of
global cooperation, the world’s nations committed themselves to stringent action to
protect the ozone layer. In September 1987 they adopted the Montreal Protocol, a
schedule to reduce and eventually eliminate the production of CFCs and halons. The
Montreal Protocol works through a system of trade barriers controlling the supply of ODS,
while permitting transfer of essential use exemptions between parties. It came into
effect 1 January 1989. The results of the Montreal Protocol have led to a
peaking of chlorinated substances in the stratosphere.
Amendments to the Protocol have:
advanced the schedule for phase-out of
chemical compounds destructive to ozone in the stratosphere;
added additional ODS to the list; and
altered requirements for essential use
exemptions.
Even as the Montreal Protocol was being
signed, it was recognized that it needed stronger controls, which were effected at the
London conference in 1990. The Protocol’s list of controlled substances was
expanded by ten, including methyl chloroform and carbon tetrachloride. Phase-out
dates were set for 2000 and 2010 for developed and developing countries,
respectively. Many parties at this conference committed themselves to the
elimination of CFCs by 1997, three years ahead of the international target. This
amendment included the provision of a fund to assist developing nations operating under
Article 5 of the Protocol. The Multilateral Fund has subsequently been assisting
developing nations to switch to more ozone-friendly substances and technology by providing
financial assistance, training and technological information. Implementing Agencies
of the Multilateral Ozone Fund include:
United Nations Environment Programme
(UNEP);
United Nations Development Programme
(UNDP);
United Nations Industrial Development
Organization (UNIDO); and
World Bank.
The 1992 Copenhagen Amendment tightened
the schedule so that developed countries were to phase out CFCs, carbon tetrachloride and
methyl chloroform by 1996 and halons by 1994. Methyl bromide and
hydrobromofluorocarbons (HBFCs) and hydrochlorofluorocarbons (HCFCs) were also added to
the list of substances to be phased out by 1996 and 2030, respectively. This
amendment also strengthened trade controls and non-compliance procedures.
The 1995 Vienna Adjustments froze the
production and consumption of methyl bromide at 1995-1998 levels and planned to freeze
consumption levels of HCFCs in 2016.
The Montreal Amendment, developed in 1997,
moved the phase-out date of methyl bromide to 2005 and 2015 for developed and developing
countries, respectively. A new licensing system involving regular exchange of
information between parties was developed to arrest illegal trade in ODS.
Initiatives of the December 1999 Beijing
Amendment, which Canada was one of the first countries to accept, has yet to be ratified
by the 20 countries required to bring it into force. It includes new controls on the
production of HCFCs, freezing production at 1989 levels in 2004 towards a phase-out in
2030 in industrialized countries, and a freeze at 2015 levels in developing countries in
2016.(59) The new amendment
also bans trade in HCFCs in 2004 with countries which have not yet ratified the 1992
Copenhagen amendment. Phase-out of a recently developed ODS, bromochloromethane, is
to be carried out in all countries by 2002. At the same time, the Executive
Committee of the Multilateral Ozone Fund decided to increase aid to India and China in
cutting production over the next ten years. These countries have had difficulty
meeting reduction targets.(60)
Imports of newly produced CFCs and halons
by developed countries have largely been banned, as have imports and exports of other
potent ODS. Existing ozone-depleting substances are re-used and recycled where
possible. Developing countries have been extended a period of grace under Article 5
of the Protocol so long as their use of ODS does not grow significantly. These
parties have kept their production of CFCs at the 1995-1997 baseline levels and are
beginning the process of phasing out the use of these substances to 50% by the year 2005.
Eastern European and large Asian nations
– such as Russia, China and India – are having difficulty incorporating advanced
refrigeration technologies. With collaboration, the much-needed changes are being
made just as these massive populations begin to fully share in this desirable
technology. Currently, China is the world’s largest producer of CFCs and
halons. The Global Environmental Facility, through UNDP, has been assisting the
phase-out of Russian CFC production facilities and the modernization of appliance
manufacturing in China(61) and India;
both of these countries are beginning to produce refrigerators which use hydrofluorocarbon
and isobutane.(62)
In 1997, the total costs of the measures
taken to protect the ozone layer were calculated to be US $235 billion. However, the
avoided adverse effects on fisheries, agriculture and materials alone are estimated to be
twice that expenditure.(63)
B. Federal
Initiatives
Canada has revised the Ozone-Depleting
Substances (ODS) Regulations, 1998, under the Canadian Environmental Protection Act,
as of January 2001, to: address the amendments to the Montreal Protocol; improve
controls on ODS; and address administrative issues.
Federal regulations prohibit production
and importation of CFC and halons in most cases, and set out strict controls on
exports. Current federal regulations prohibit import and export of recovered halons
except by permit.
Restrictions on the refilling of equipment
encourage conversion to alternatives. The transition is made easier due to the
imposition of decreased supply so that the cost of CFCs is increasing steadily while the
cost of replacements is decreasing. Canadian ODS reduction is apparent in the
following figure.
Figure 4 (64)
CANADIAN ODS CONSUMPTION
(kilotonnes)

In 1996, manufacturers started using
alternative refrigerants for new equipment. However, a large number of
CFC-containing units remained available on the market until recently. These
appliances have a long service life of approximately 13-15 years. The stock of CFCs
should be available for servicing household appliances until 2020.
Production of carbon tetrachloride, methyl
chloroform, and methyl bromide has been phased out in industrialized nations and there is
not a serious surplus to manage in Canada.
At this time, Canada has one permanent
approved incineration facility for disposal of ODS, located at Swan Hills, Alberta.
Because the facility has limited capacity, Canada may allow the export of ODS for
destruction at a suitable facility abroad. Currently, other facilities capable of
destroying ODS are operating in Australia, Sweden, Germany, Japan, the United Kingdom,
Russia and the United States.
To permit Canadians to take suitable
precautions against increased UV-B levels, Environment Canada announced an advisory
service shortly after NASA had confirmed the strong possibility of a substantial reduction
of the ozone layer over a large area of Canada. The service began 13 March 1992,
much sooner than originally planned, and provides daily information on guarding against
exposure to the sun in view of changing UV-B levels, making Canada the first country to
issue nation-wide daily forecasts of solar UV radiation. The UV Index has been
emulated around the world, allowing the public to limit their exposure when the harmful
rays are most intense.(65)
A cross-country network of monitoring
stations has kept continuous watch on Canada’s ozone layer for more than three
decades. The existence of these early records, before any major human influence on
the upper atmosphere, is vital to understanding the changes that have occurred. The
World Ozone and Ultraviolet Radiation Data Centre (WOUDC) is one of six recognized World
Data Centres which are part of the Global Atmosphere Watch (GAW) programme which in turn
is part of the World Meteorological Organization (WMO). The WOUDC, operated by the
Experimental Studies Division of the Meteorological Service of Canada,(66) is located in Toronto.
C. Federal-Provincial
Action
International agreements have been
translated into national objectives. In Canada, the federal government has been
working very cooperatively with the provinces to implement the necessary changes.
Industry has also become increasingly involved. The Canadian Council of Ministers of
the Environment (CCME) directed in April 1989 that the Federal-Provincial Action Committee
(FPAC) of the Canadian Environmental Protection Act coordinate the development of
controls across all jurisdictions. The CCME has taken the lead in organizing
multi-jurisdictional participation in the reduction, recovery and recycling of CFCs.
On 21 August 1990, the CCME established a working group to develop a National Action
Plan for reduction, recovery and recycling.
The CCME accepted and published the
National Action Plan in 1992. The rapid development of such a major plan
demonstrates the level of cooperation between the various governments on this important
environmental issue. This plan identified six major tasks, which have been completed
or are ongoing:
1. To mandate, under provincial
regulations, the recovery, recycling and reclamation of CFCs and HCFCs from all
refrigeration and air conditioning uses. This includes banning the deliberate
release of CFCs and HCFCs into the atmosphere.
2. To develop, in conjunction with
industry trade associations, training programs in recovery and recycling for the service
sector. The training would include the Federal Code of Practice for Emission
Reduction of CFCs, as well as hands-on equipment training.
3. To characterize the existing bank
of CFCs in Canada, necessary to measure the effectiveness of recovery/recycling activities
and to plan for ultimate destruction scenarios.
4. To develop, with the involvement
of industry and standards associations, appropriate standards for the quality of recycled
refrigerant and for the performance of recovery equipment.
5. To ensure that the general public
is informed of the problem and the solutions. The public’s reaction and
participation is an integral part of resolving the problem.
6. To revise government
purchasing/procurement standards, including service contracts, to ensure recovery and
recycling of CFCs, HCFCs and halons.
The most recent National Action Plan
for the Environmental Control of Ozone-Depleting Substances and their Halocarbon
Alternatives (NAP), published in 1998 by the Canadian Council of Ministers of the
Environment, aims to continue to reduce emissions of ozone-depleting substances.
In 1998, NAP stated the following
objectives:
To improve the environmental management
of all ODS and halocarbon alternatives and to reduce their emissions during the
installation, operation, maintenance, repair, disposal, and decommissioning of systems and
equipment.
To provide consistency for industry and
to minimize the impact on other environmental issues.
To meet these new needs by revising the
original tasks, including:
- the continuation of original tasks
as needed;
- original tasks that have been
expanded to include halocarbon alternatives;
- new tasks to reflect the revised
“Environmental Code of Practice for Elimination of Fluorocarbon Emissions from
Refrigeration and Air Conditioning Systems” and to enhance the recovery of
refrigerants;
- new tasks reflecting the “Code
of Practice for halons”;
- new tasks focused on other industry
sectors to reduce fluorocarbon emission; and
- new tasks to provide basic
information necessary for controlling future actions.
All provincial governments and one
territorial government have regulations controlling the release of ODS and mandating
recovery and recycling.(67)
D. Action Still to be
Taken
At the most recent meeting of the parties,
in Beijing, the Executive Secretary of the Ozone Secretariat, K. Madhava Sarma, noted that
consumption and production of ODS has fallen by nearly 90% but that there are hurdles to
overcome. These were listed as growing emissions due to:
exemptions;
increased global warming which could
delay the ozone layer’s recovery;
new ozone-depleting substances coming
onto the market; and
slow ratification of the Protocol’s
amendments, illustrated in Figure 5.(68)
Figure 5
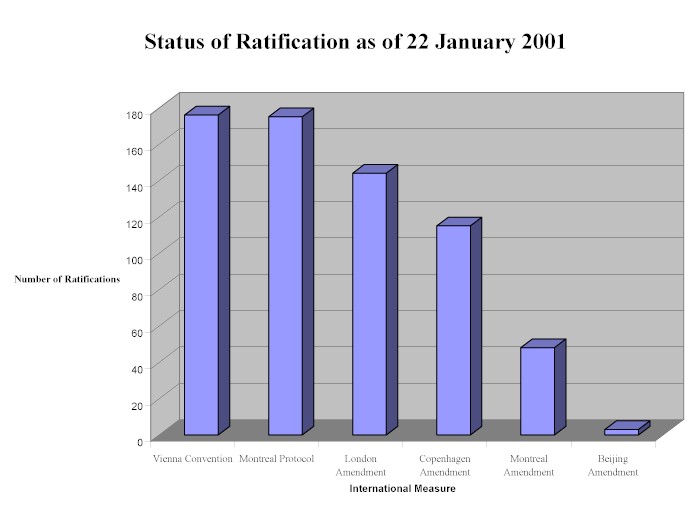
As previously mentioned, HCFCs – the
current replacements for CFCs – are scheduled to be phased out in the next few
decades due to their high greenhouse gas potential, so that replacements for these, too,
will be needed.
Vast inventories of equipment and
materials containing CFCs still exist. The phase-out continues for developed
nations, which have been using CFCs since they were invented more than 50 years
ago. If ODS are not successfully phased out of the growing economies of Asia, in
particular, the Protocol will not succeed.
Much of the foam and insulation containing
CFCs are in refuse piles with liberation of these ODS remaining uncertain. In
addition, the world has a billion refrigeration and air conditioning units, many of which
are poorly maintained and leaking CFCs, and which, when they are no longer serviceable,
are simply thrown into landfill sites. These vast quantities of CFCs may still find
their way into the atmosphere.
As a result of the decline in the
production and use of CFCs in developed countries and the continuation of CFC production
in developing countries until 2010, lucrative international smuggling of CFCs exists.(69) The extent of the smuggling
is an estimated 10% of the legal trade.(70)
Parties of the Montreal Protocol have been urged at meetings to install
licensing programs and to subject smugglers to heavy penalties. The United States
currently has the largest black market of ODS.(71) To address the issue of smuggling, surveyed
countries suggested the following actions:
drafting legislation;
coordinating agencies;
implementing a system of licensing; and
training prosecutors, investigative
agents and customs officials.(72)
It has recently been understood that
although “greenhouse gases” are warming the atmosphere near the Earth’s
surface, they are also cooling the stratosphere by altering the radiative balance and
consequently strengthening the Arctic polar vortex.(73) It must be recognized that global
warming is inter-connected with ozone depletion in the stratosphere. In February of
1999, Hartmut Grassl, the director of the UN’s World Climate Research Programme, made
the upper atmosphere his new number one priority in the wake of concern that not enough is
known about changes in the upper atmosphere above inhabited polar regions of the North.(74)
SUMMARY AND
CONCLUSION
Life on Earth remains a delicate balance
of many different elements. Use of ozone-depleting substances has jeopardized what
acts as the Earth’s sunscreen. The harmful effects of increased UV-B have been
worsening. Now that research is confirming a connection between climate change and
depletion of the ozone layer, predictions for recovery have been extended.(75)
The international community has cooperated
as never before to remedy this situation. The reduction, recycling and replacement
of ozone-destroying substances is progressing. Thus far, industrialized countries
have reduced production and consumption of ODS by 85%.(76) New technologies are being developed so that
ozone-depleting agents can be eliminated. Although the concentration of chlorine is
declining in the stratosphere and the rate of increase of halons is slowing, several
models now show the peak of ozone depletion occurring around the year 2020, rather than
the previous estimate of the year 2000.(77)
Had there not been international effort to limit the amount of ozone-depleting chemicals
in the atmosphere, the situation would be much worse, and would have continued for many
more decades.
If international commitments are kept, the
ozone layer is expected to recover to pre-1980 levels during the latter half of this
century.(78) With the
intricacies and inter-relationships of ozone chemistry and mechanics of the upper
atmosphere unfolding, the global community’s full compliance with the Montreal
Protocol and with efforts to control greenhouse gases is proving necessary in regaining
the natural balance between ozone production and destruction in the upper atmosphere.
Appendix
Ozone-Depleting
Substances
Chemical
Name |
Lifetime,
in years |
ODP |
CFC-11 (CCl3F)
Trichlorofluoromethane |
45 |
1.0 |
CFC-12 (CCl2F2)
Dichlorodifluoromethane |
100 |
1.0 |
CFC-113 (C2F3Cl3)
1,1,2-Trichlorotrifluoroethane |
85 |
0.8 |
CFC-114 (C2F4Cl2)
Dichlorotetrafluoroethane |
300 |
1.0 |
CFC-115 (C2F5Cl)
Monochloropentafluoroethane |
1700 |
0.6 |
Halon 1211 (CF2ClBr)
Bromochlorodifluoromethane |
11 |
3.0 |
Halon 1301 (CF3Br)
Bromotrifluoromethane |
65 |
10.0 |
Halon 2402 (C2F4Br2)
Dibromotetrafluoroethane |
|
6.0 |
CFC-13 (CF3Cl)
Chlorotrifluoromethane |
640 |
1.0 |
CFC-111 (C2FCl5)
Pentachlorofluoroethane |
|
1.0 |
CFC-112 (C2F2Cl4)
Tetrachlorodifluoroethane |
|
1.0 |
CFC-111 (C3FCl7)
Heptachlorofluoropropane |
|
1.0 |
CFC-112 (C3F2Cl6)
Hexachlorodifluoropropane |
|
1.0 |
CFC-113 (C3F3Cl5)
Pentachlorotrifluoropropane |
|
1.0 |
CFC-114 (C3F4Cl4)
Tetrachlorotetrafluoropropane |
|
1.0 |
CFC-115 (C3F5Cl3)
Trichloropentafluoropropane |
|
1.0 |
CFC-116 (C3F6Cl2)
Dichlorohexafluoropropane |
|
1.0 |
CFC-117 (C3F7Cl)
Chloroheptafluoropropane |
|
1.0 |
CC14 Carbon tetrachloride |
35 |
1.1 |
Methyl Chloroform
(C2H3Cl3)
1,1,1-trichloroethane |
4.8 |
0.1 |
Methyl Bromide (CH3Br) |
0.7 |
|
CHFBr2 |
|
1.0 |
HBFC-12B1 (CHF2Br) |
|
0.74 |
CH2FBr |
|
0.73 |
C2HFBr4 |
|
0.3
– 0.8 |
C2HF2Br3 |
|
0.5
– 1.8 |
C2HF3Br2 |
|
0.4
– 1.6 |
C2HF4Br |
|
0.7
– 1.2 |
C2H2FBr3 |
|
0.1
– 1.1 |
C2H2F2Br2 |
|
0.2
– 1.5 |
C2H2F3Br |
|
0.7
– 1.6 |
C2H3FBr2 |
|
0.1
– 1.7 |
C2H3F2Br |
|
0.2
– 1.1 |
C2H4 Br |
|
0.07
– 0.1 |
C3HFBr6 |
|
0.3
– 1.5 |
C3HF2Br5 |
|
0.2
– 1.9 |
C3HF3Br4 |
|
0.3
– 1.8 |
C3HF4Br3 |
|
0.5
– 2.2 |
C3HF5Br2 |
|
0.9
– 2.0 |
C3HF6Br |
|
0.7
– 3.3 |
C3H2FBr5 |
|
0.1
– 1.9 |
C3H2F2Br4 |
|
0.2
– 2.1 |
C3H2F3Br3 |
|
0.2
– 5.6 |
C3H2F4Br2 |
|
0.3
– 7.5 |
C3H2F5Br |
|
0.9
– 1.4 |
C3H3FBr4 |
|
0.08
– 1.9 |
C3H3F2Br3 |
|
0.1
– 3.1 |
C3H3F3Br2 |
|
0.1
– 2.5 |
C3H3F4Br |
|
0.3
– 4.4 |
C3H4FBr3 |
|
0.03
– 0.3 |
C3H4F2Br2 |
|
0.1
– 1.0 |
C3H4F3Br |
|
0.07
– 0.8 |
C3H5FBr2 |
|
0.04
– 0.4 |
C3H5F2Br |
|
0.07
– 0.8 |
C3H6FBr |
|
0.02
– 0.7 |
HCFC-12 (CHF2Cl)
Monochlorodifluoromethane |
11.8 |
0.055 |
HCFC-123 (C2HF3Cl2)
Dichlorotrifluoroethane |
1.4 |
0.02 |
HCFC-124 (C2HF4Cl)
Monochlorotetrafluoroethane |
6.1 |
0.022 |
HCFC-141b (C2H3FCl2)
Dichlorofluoroethane |
9.2 |
0.11 |
HCFC-142b (C2H3F2Cl)
Monochlorodifluoroethane |
18.5 |
0.065 |
HCFC-225ca (C3HF5Cl2)
Dichloropentafluoropropane |
2.1 |
0.025 |
HCFC-225cb (C3HF5Cl2)
Dichloropentafluoropropane |
6.2 |
0.033 |
Source: United
States Environmental Protection Agency, Global Programs Division, October 2000
Original Sources:
Figures in the Lifetime column are from Table 10-8 of the Scientific
Assessment of Ozone Depletion, 1998, a report of the World Meteorological
Organization’s Global Ozone Research and Monitoring Project.
Figures in the ODP column are from Table 11-1 of the Scientific
Assessment of Ozone Depletion. Blanks in the data indicate
that the information was not shown in the original source.
(1)
National Aeronautics & Space Administration, Ames Research Center,
NASA News, April 2000.
(2) Environment
Canada, Arctic Ozone: The Sensitivity of the Ozone Layer to
Chemical Depletion and Climate Change, December 1998.
(3) Environment
Canada, Atmospheric Climate Science Directorate, “Stratospheric
Ozone,” January 2001.
(4)
Paul A. Newman, SAGE III Ozone Loss and Validation Experiment, SOLVE:
A NASA DC-8, ER-2 and High Altitude Balloon Mission, National Aeronautics
& Space Administration/Goddard Space Flight Center, March 1999.
(5)
Paul J. Crutzen and Veerabhadran Ramanathan, “Pathways of Discovery
– The Ascent of Atmospheric Sciences,” Science, Vol.
290, 13 October 2000.
(6)
United Nations Environment Programme (UNEP), The Ozone Secretariat,
Frequently Asked Questions About Ozone to the Scientific Assessment
Panel, 2000.
(7)
Catalysts are characterized by the same quantity being present at the
beginning and end of a reaction.
(8)
The general term for chlorofluorocarbons (CFCs), and other substances
of similar composition containing bromine.
(9)
UNEP, Frequently Asked Questions About Ozone to the Scientific
Assessment Panel, 2000.
(10)
Thomas G. Chasteen, Ozone’s Problem with Polar Stratospheric
Clouds, Dept. of Chemistry, Sam Houston State University, Spring
1997.
(11)
Charged particles.
(12)
Ibid.
(13)
Newman, SAGE III Ozone Loss and Validation Experiment, 1999.
(14)
Break apart due to ultraviolet radiation.
(15)
Richard A. Kerr, “Stratospheric ‘Rocks’ May Bode Ill
for Ozone,” Science, Vol. 291, No. 5506, February 2001.
(16)
Christiane Voigt et al., “Nitric Acid Trihydrate (NAT) in
Polar Stratospheric Clouds,” Science,Vol. 290, No.
5497, October 2000.
(17)
Ibid.
(18)
Environment Canada, Stratospheric Ozone, January 2001.
(19)
Environment Canada, Arctic Ozone, 1998.
(20)
UNEP, State of the Environment Norway, GRID-Adrendal, Original source:
UNEP/ (GEMS) library series no 7: The Impact of Ozone Layer
Depletion, May 1997.
(21)
Ibid.
(22)
World Health Organization, “The Global UV Project Summary,”
Intersun, October 1998.
(23)
“11th MOP to the Montreal Protocol and 5th
COP to the Vienna Convention,” Linkages, December 1999.
(24)
UNEP, State of the Environment Norway, 1997.
(25)
World Health Organization, “The Global UV Project Summary,”
1998.
(26)
Environmental Effects of Ozone Depletion: 1991 Update (1991),
p. iii.
(27)
Synthesis of the Reports of the Ozone Scientific Assessment Panel, Environmental
Effects Assessment Panel, Technology and Economic Assessment Panel,
Prepared by the Assessment Chairs for the Parties to the Montreal Protocol,
November 1991, p. 6.
(28)
Ibid., p. iv.
(29)
Crutzen and Ramanathan, “Pathways of Discovery,” 2000.
(30)
Fred Pearce, “Algal Gloom,” New Scientist, August 1998.
(31)
Stephen O. Anderson, “Halons and the Stratospheric Ozone Issue,”
Fire Journal, Vol. 8, No. 3, May-June 1987.
(32)
Reinhard Pienitz and Warwick F. Vincent, “Effect of climate change
relative to ozone depletion on UV exposure in sub arctic lakes,”
Nature, pp. 404, 484-487, March 2000.
(33)
Containing carbon.
(34)
UNEP, State of the Environment Norway, 1997.
(35)
The 2000 ozone hole, British Antarctic Survey, December 2000.
(36)
UNEP, Frequently Asked Questions About Ozone to the Scientific Assessment
Panel, 2000.
(37)
Environment Canada, The Canadian Middle Atmosphere Initiative, April
2000.
(38)
Canadian Space Agency, SCISAT-1: Canada’s next scientific satellite,
Apogee – Space Science, October 1999.
(39)
Environment Canada, Arctic Ozone, 1998.
(40)
Fred Pearce, “Chill in the air,” New Scientist, May
1999.
(41)
Joyce E. Penner et al.,“Aviation and the Global Atmosphere
Summary for Policy Makers,” Intergovernmental Panel on Climate
Change, United Nations Environment Programme/World Meteorological Organization,
April 1999.
(42)
“Summary of the Eleventh Meeting of the Parties to the Montreal
Protocol and the Fifth Conference of the Parties to the Vienna Convention,”
Earth Negotiations Bulletin, Vol. 19, No. 6, December 1999.
(43)
“Supersonic Aircraft Exhaust Measurements to Help Ozone Aircraft
Studies,” NASA HQ Public Affairs Office, October 1995.
(44)
S.A. Montzka, J.H. Butler, J.W. Elkins, T.M. Tompson, A.D. Clarke
and L.T. Lock, “Present and future trends in the atmospheric burden
of ozone-depleting halogens,” Nature, Vol. 398, April
1999.
(45)
Environment Canada, “Success Stories – Finding Alternatives,”
The Green Lane, 1997.
(46)
A stationary wave formed of several identical waves overlapping.
(47)
The region of maximum amplitude between two adjacent nodes in a standing
wave.
(48)
“Cooling with Sound: An Effort to Save Ozone Shield,”
New York Times, 25 February 1992.
(49)
Peter T. Landsberg, “Thermodynamics: Cool Sounds,” Nature,
August 1998.
(50)
Mark Alpert, “A Cool Idea,” Scientific American, May
1998.
(51)
Naomi Lubick, “Desert Fridge,” Scientific American, November
2000.
(52)
Alan Hall, “What’s the Beef?” Scientific American,
November 1997.
(53)
Ammonia Refrigeration, Occupational Safety & Health Administration,
U.S. Department of Labor, March 2001
(54)
William Murray, “New Technology Developments as a Result of CFC
Phase-Out,” Prepared for the House of Commons Standing Committee
on Environment, Parliamentary Research Branch, Library of Parliament,
1 April 1992, p. 2.
(55)
“Phasing Out CFC-Inhalers – Making the Transition to CFC-free
MDIs,” The Green Lane, Environment Canada, July 1999.
(56)
“No More Ozone-Depleting Solvents,” Toronto Star, 16 December
1991.
(57)
Environment Canada, “Success Stories,” 1997.
(58)
Also known as ozone-depleting chemicals (ODCs).
(59)
Environment News Service, “Stronger Global Ozone Layer Action Agreed,”
December 1999.
(60)
Ehsan Masood, “Ozone recovery will be long-term affair,” Nature,
Vol. 373, June 1998.
(61)
Environment News Service, “China Warms to Fridge Project,”
December 1999.
(62)
Namrata Singh, “Godrej-GE Appliances to phase out CFC by 2000,”
The Financial Express, India, April 1998.
(63)
UNEP, Frequently Asked Questions About Ozone to the Scientific Assessment
Panel, 2000.
(64)
Canadian Council of Ministers of the Environment, National Action
Plan, Environmental Protection Service of Environment Canada, January
1998.
(65)
Environment Canada, “The health impacts of living with ultraviolet
radiation,” September 1997.
(66)
Formerly Atmospheric Environment Services.
(67)
Canadian Council of Ministers of the Environment, National Action
Plan, Environmental Protection Service of Environment Canada, January
1998.
(68)
“Summary of the 20th Meeting of the Open-ended Working
Group of the Parties to the Montreal Protocol on Substances that Deplete
the Ozone Layer: 11-13 July 2000,” Earth Negotiations
Bulletin, Vol. 19, No. 7, December 2000.
(69)
David Spurgeon, “Ozone treaty ‘must tackle CFC smuggling’,”
Nature, Vol. 389, No. 219, September 1997.
(70)
Vinod Chhabra, “Montreal Protocol and After,” The Financial
Express, India, August 1997.
(71)
“11th MOP to the Montreal Protocol and 5th
COP to the Vienna Convention,” Linkages, December 1999.
(72)
Ibid.
(73)
Drew T. Shindell, David Rind and Patrick Lonergan, “Increased polar
stratospheric ozone losses and delayed eventual recovery owing to increasing
greenhouse-gas concentrations,” Nature, April 1998.
(74)
Pearce, “Chill in the air,” 1999.
(75)
Peter Aldhous, “Global warming could be bad news for Arctic ozone
layer,” Nature, Vol. 404, April 2000.
(76)
“11th MOP to the Montreal Protocol and 5th
COP to the Vienna Convention,” Linkages, December 1999.
(77)
Shindell, Rind and Lonergan, “Increased polar stratospheric ozone
losses and delayed eventual recovery owing to increasing greenhouse-gas
concentrations,” 1998.
(78)
R. Monastersky, “A sign of healing appears in stratosphere,”
Science News, 18 December 1999.
|